Peptide depots and DNA tattoos could deliver drugs in the future
New methods boost the efficiency of biologically-inspired treatments.
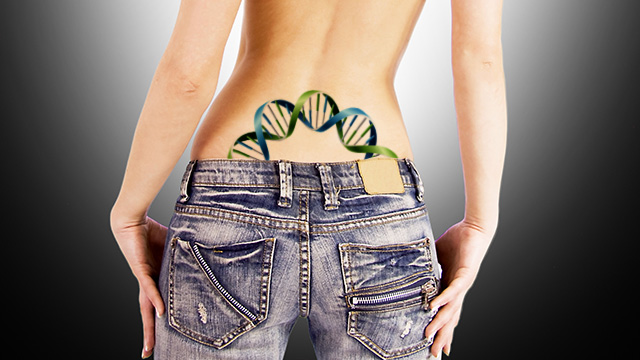
Most of these, however, haven't always worked out well in the real world. Proteins need to be injected to avoid the digestive system, but are often cleared from the blood quickly enough that repeated injections are needed. DNA vaccines work great, provided you can get the DNA into cells, which has turned out to be a significant technical hurdle.
Two papers, published this week, provide some indications of how researchers are working to get around these hurdles.
Meet the POD
The classic example of a disease treated by protein injections is type 1 diabetes. In this disorder, the cells that normally produce insulin are destroyed by the immune system. Regular injections of the protein, now made in bacteria, can keep levels in the blood stable. This treatment has made diabetes a manageable disease, at least in the developed world.But protein injections have their problems. The proteins need to be kept refrigerated or they'll degrade. Insulin pills won't work since the digestive system will destroy the protein. And, because the body clears insulin from the blood at an appreciable rate, frequent monitoring and injection are needed to maintain reasonable levels.
The authors of a new paper were interested in using glucagon-like peptide-1 therapeutically (GLP-1). The small protein hormone helps regulate glucose levels, but is incapable of boosting expression to levels where it becomes harmful. Unfortunately, injected GLP-1 is also cleared from the blood so quickly that its half life is about two minutes. Injections at a rate fast enough to maintain levels simply aren't possible.
So, the researchers decided to set up a slow-release system. They created a gene that was essentially a string of GLP-1 sequences, separated by sites recognized by one of the body's protein cutting enzymes. Then they linked these to a sequence for something called elastin-like peptide, or ELP. ELP has an interesting behavior: it's perfectly soluble in water at room temperature, but forms a complex aggregate when raised to human body temperatures.
The proteins were made in bacteria, then purified by raising the temperature—the ELP aggregated out of solution, separating it from the other bacterial proteins. Lower the temperature, and it went back into solution; inject the solution under the skin, and it would form another aggregate.
The authors called the resulting aggregate a POD, for Protease Operated Depot. Over several days, the body's protein cutting enzymes (proteases) would gradually chew up the aggregate, slowly releasing the GLP-1 in the process. The authors found that a single POD injection could keep the peptide levels in the blood stream of a test animal stable for up to five days. And they suggest that, by varying the sites that triggered the GLP-1 release, they could stretch that out even longer.
Tattooed vaccines
DNA vaccines are another great idea that hasn't worked out yet. Instead of using an inactivated pathogen or purified proteins, you can just inject DNA that encodes some of the pathogen's proteins. Any cells that pick up the DNA will make the foreign proteins, triggering a robust immune response. The problem, however, has been getting enough cells to pick up the DNA. Most DNA floating around outside of cells gets treated like a threat and digested.One possible answer to this problem may come in the form of something akin to a DNA-containing tattoo. The authors created a layered polymer structure that will slowly degrade at the pH normally found in the human body. Some of the layers are simply structural, but others contain a mix of the DNA for the vaccine and chemicals that help get the DNA inside cells.
They were able to form this polymer as a layer on top of a grid of many tiny microneedles. Each one is much smaller than the needles normally used for injection, but still capable of penetrating the outer layers of the skin and depositing the polymer beneath it, in much the same way that a tattooing needle deposits ink underneath the skin. Once injected, the polymer slowly degraded, gradually releasing DNA that was taken up by nearby cells.
The authors didn't test this technique with a vaccine, but they did show that cells of the skin take up DNA and express any proteins it encodes. So, the general principle seems to be sound. And, just like the POD developers, this paper's authors suggest that the material properties of the injection could be tuned to adjust the timing and strength of the DNA release. Plus, the polymers are stable for extended periods at room temperature.
We're still a long way off from seeing these technologies in the doctor's office. But it's nice to see that people are coming up with clever ideas to overcome some of the difficulties that have limited the impact of biotechnology.
PNAS, 2013. DOI: 10.1073/pnas.1214518110, Nature Materials, 2013. DOI: 10.1038/NMAT3550 (About DOIs).
No comments:
Post a Comment